Demystifying Dust Explosion Propagation for an Overall Explosion Protection Strategy
The unfortunate propensity of dust explosions to destroy entire facilities and claim lives has been reported in numerous past incidents. A recent illustration is the massive explosion that occurred on February 7th, 2008 at the Imperial Sugar Company in Port Wentworth (Georgia), where 14 people were killed and 36 people were injured.
Powder handling processes are often comprised of interconnected enclosures and equipment. Flame and pressure resulting from a dust explosion can therefore propagate through piping, across galleries, and reach other pieces of equipment or enclosures, leading to extensive damage.
While the ability of dust explosions to propagate has been widely recognized, some misconceptions lead to the false sense of security that explosion isolation is not required.
Resolving Common Misconceptions About Explosion Propagation
Inspired by Paul Amyotte’s book “An Introduction to Dust Explosions” [1], this article will enumerate, illustrate and unravel the following common myths about explosion propagation:
Myth #1: | Myth #2: | Myth #3: | Myth #4: | Myth #5: | |
---|---|---|---|---|---|
a large amount of dust is needed for an explosion to propagate |
a dust explosion starting in a vented vessel cannot propagate through connected pipes |
a dust explosion cannot propagate against process flow |
a dust explosion weakens as it propagates |
small diameter pipes do not support dust explosion propagation |
myth
|
A Large Amount of Dust is Needed for An Explosion to Propagate |
fact |
Dust Explosions Do Not Need Large Amounts of Fuel to Propagate |
Even a 1 mm layer can create a dust explosion hazard
In his book [2], Eckhoff underlines that even a 1 mm layer can create a dust explosion hazard in a typical room. This has been confirmed experimentally by Tamanini [3], who carried out a series of cornstarch explosion tests in a full-scale gallery equipped with several vent panels. The explosion only needed a 1/100 inch layer of dust on the ground to fully propagate.
During a vented explosion, unburned fuel is ejected outside the primary enclosure and is able to create a secondary explosion. The same phenomenon happens when a dust explosion occurs in a vessel connected to pipes. Valiulis et al. [4] studied the flame propagation into an 89 foot long clean air duct connected to a 0.64 m3 initiating enclosure containing cornstarch or phenolic dust. Tests conducted have resulted in flame propagation distances in the range of 22-89 feet, even in a clean air duct.
Recent findings from the Fike Corporation’s research on dust explosion propagation [5, 6] have shown that a flame propagates even more easily when dust concentration into the pipes is low (50 g/m3 compared to 500 g/m3), both for low reactivity (wood flour) and high reactivity (phenolic dust) fuels; this was attributed to the increased inertia of richer dust-air mixtures.
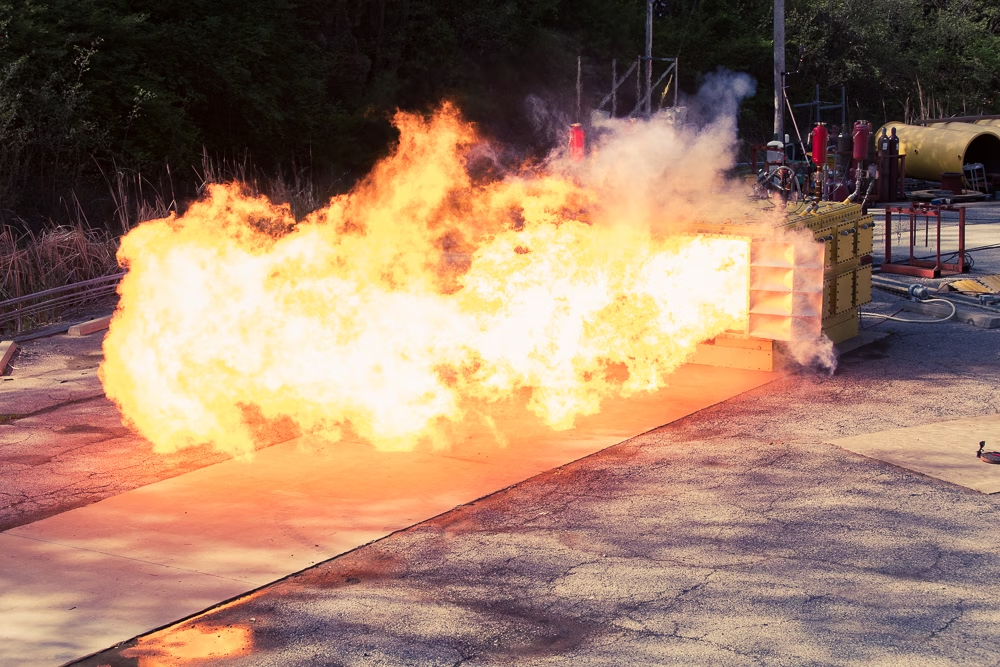
myth
|
A Dust Explosion Starting in a Vented Vessel Cannot Propagate Through Connected Pipes |
fact |
Venting Does Offer Protection, but it Does Not Necessarily Prevent Explosion Propagation |
Explosion in a primary vented vessel can propagate to a secondary vented vessel
It is a common belief that protecting an enclosure, by means of venting or suppression, will affect explosion propagation in such a manner that no explosion isolation is needed at all.
In the early 1990’s, Chatrathi et al. [7] performed gas explosion experiments using a 2.6 m3 vessel connected to pipes, and were able to provide evidence that “[…] although venting protects a vessel from the high pressures generated by an explosion, it does not necessarily prevent the explosion from being propagated through piping into other vessels.” This statement is also fully valid for dust explosions.
Van Wingerden et al. [8] carried out a series of tests with maize starch, peat and wheat dusts to study explosion propagation in an integrated system including a 5.8 m3 vented bag filter, connected to a 2 m3 vessel. Experiments with maize starch resulted in explosion propagation through the entire system.
Holbrow et al. [9] conducted explosions tests with coal, toner and anthraquinone dusts using a combination of vented vessels (2 m3, 6.3 m3, 20 m3), connected by a pipe up to 49 feet long. They showed that an explosion in a primary vented vessel can propagate to a secondary vented vessel (Figure 1).
Figure 1. Interconnected vented vessels explosion tests performed by Health and Safety Laboratory (UK)
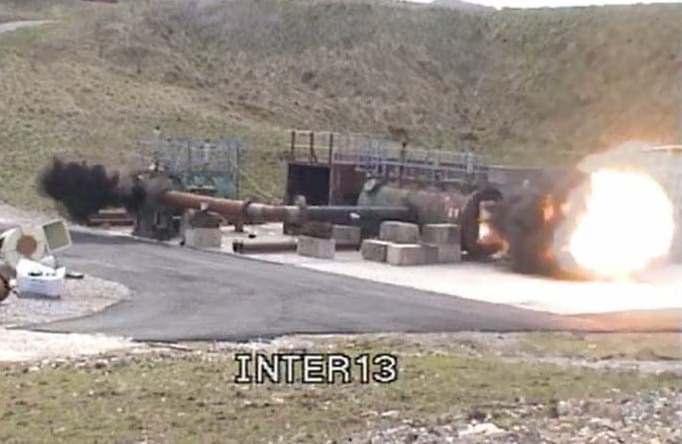
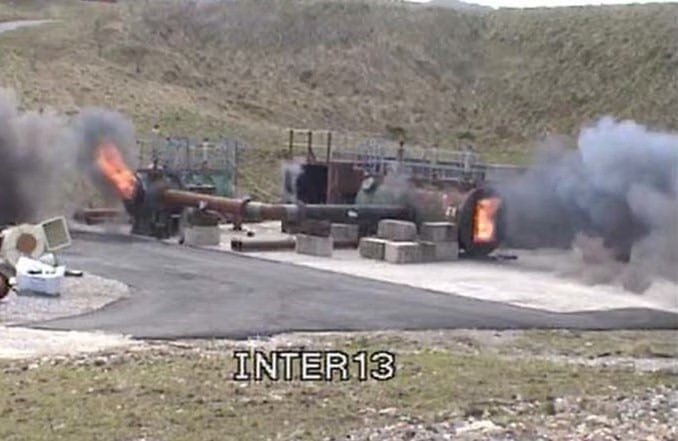
Fike Corporation Remote Test Site
Fike Corporation routinely performs demonstration tests at its remote testing facility. One of these tests involves a dust collector equipped with two pipes. On Figure 2, one can see that even though the dust collector is vented, flame can propagate through these pipes. Ultimately, chemical isolation with sodium bicarbonate (blue cloud on the right of the figure) is needed to extinguish the resulting flame.
Figure 2. Demonstration tests performed by Fike Corporation (USA)
The fact that a dust explosion from a vented vessel can propagate through pipes over long distances is also well illustrated by the incident that occurred on October 29th, 2003 in Huntington, Indiana, USA (Figure 3, below). In this plant manufacturing automotive wheels, an aluminum dust explosion started in a dust collector protected by a vent panel and propagated through pipes to the entire facility, resulting in 1 fatality and 7 injuries [10].
Figure 3. View of the dust collector (outside)
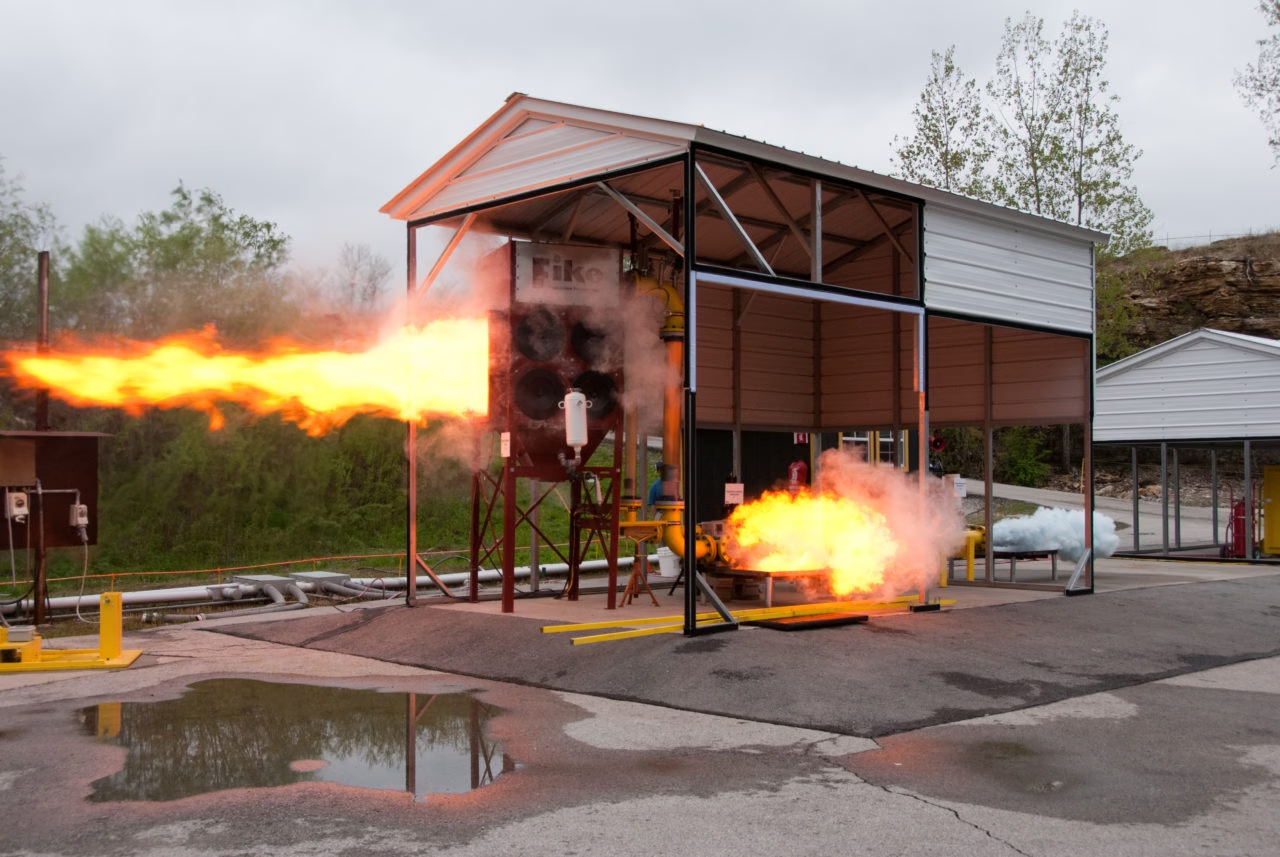
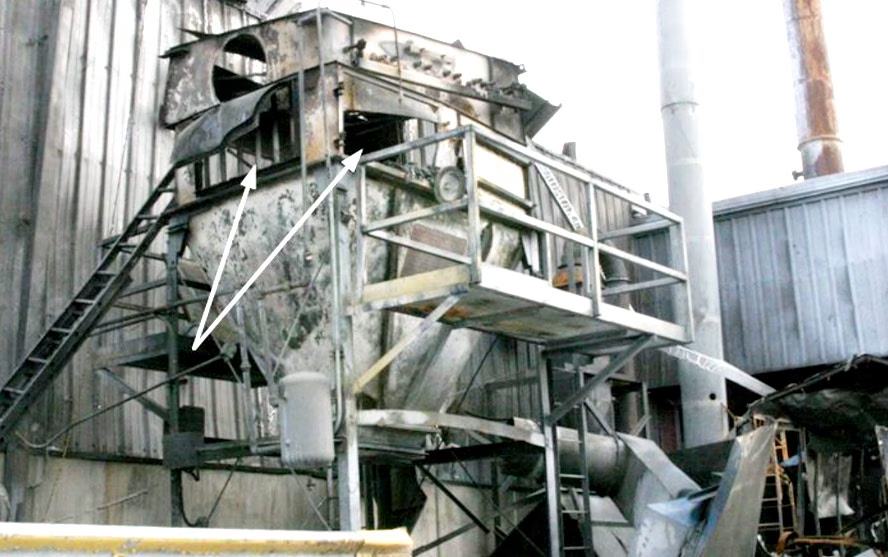
myth
|
A Dust Explosion Cannot Propagate Against Process Flow |
fact |
An Explosion is Capable of Traveling Both with and Against Process Flow |
Explosion Propagation Tests in a Pneumatic Conveying System
An argument also often heard is that a dust explosion cannot propagate against pneumatic process flow.
To challenge this statement, Vogl [11] conducted explosion propagation tests in a pneumatic conveying system consisting of a dust feeder, a 131 foot (or 157 foot) conveying pipe, a cyclone and a suction fan. Maize starch, lycopodium, and wheat flour were used as fuels. Dust concentration into the pipe was varied in the range 75 - 450 g/m3. Further work by the same authors [12] included a vented initiating vessel. Both experimental programs clearly demonstrated that an explosion is capable of traveling both with and against process flow (Figure 4), even over long distances.
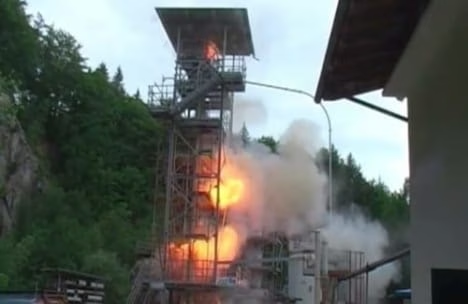
Figure 4. Dust explosion propagation tests performed by FSA
(Germany)
Fike Corporation Dust Explosion Testing
More recently [5, 6], Fike Corporation conducted an extensive experimental program to study dust explosion propagation in industrial conveyance systems. For that purpose, a large- scale test rig comprising of a vented vessel connected to two pipelines was erected at Fike Corporation’s remote testing facility. By means of a fan, different flow conditions were established (from 15 m/s to 30 m/s).
Among other findings, this research ultimately showed that explosion propagation from a conveyed vented vessel is possible both with and against process flow (Figure 5).
Figure 5. Dust explosion propagation tests (active conveyance) performed by Fike Corporation (USA)
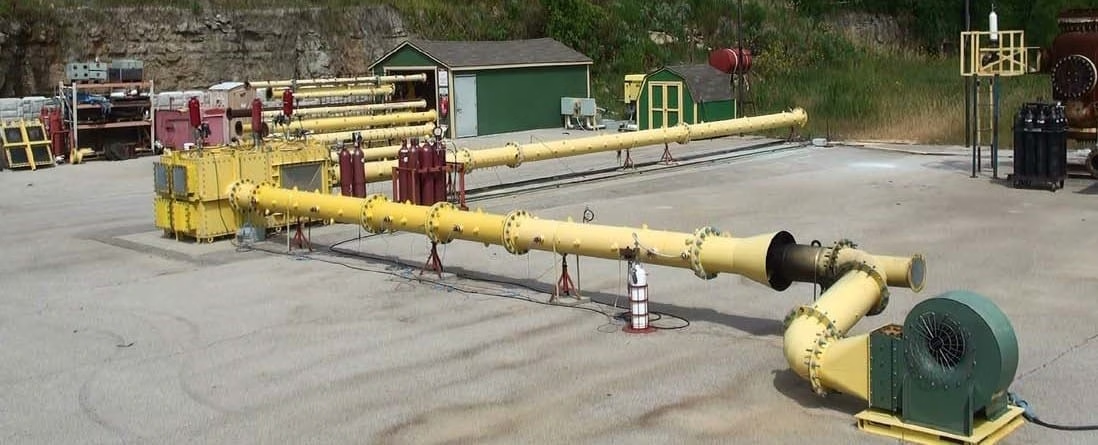
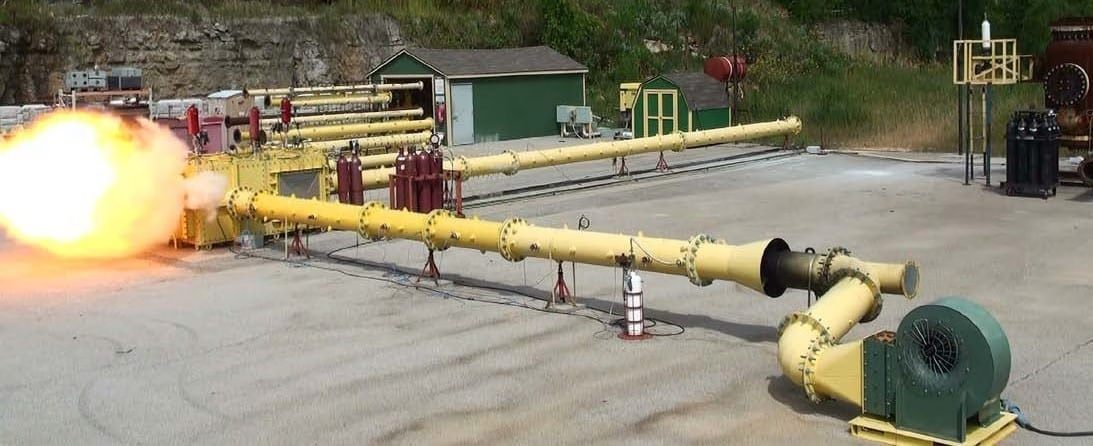
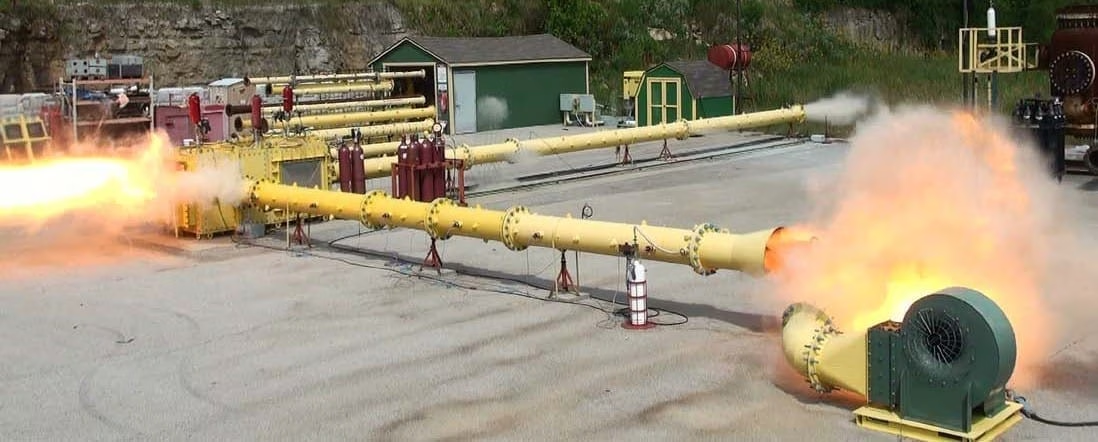
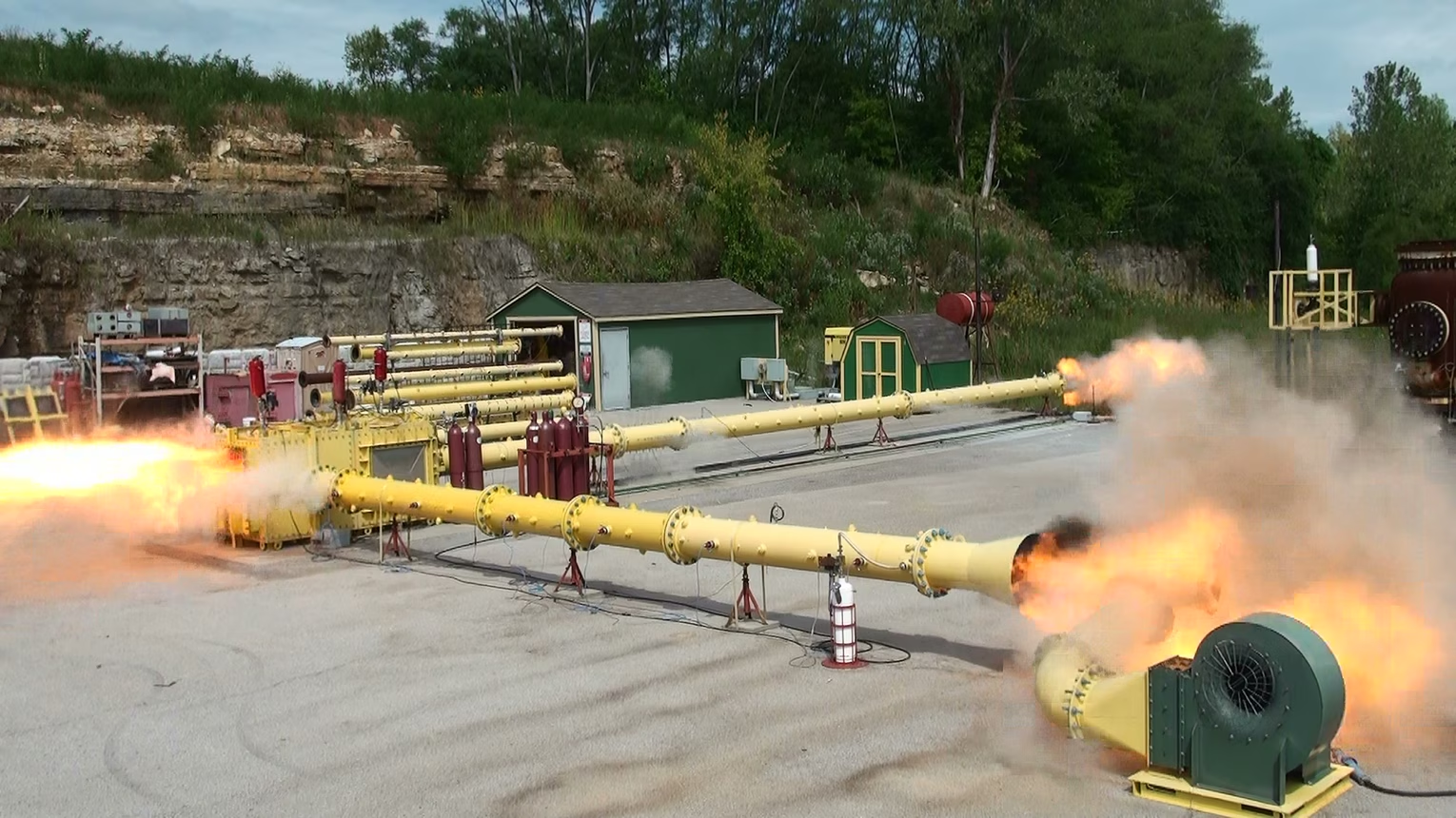
myth
|
A Dust Explosion Weakens as it Propagates |
fact |
Explosions Propagate, and Become Increasingly More Damaging |
Explosion & Interconnected Vessels
Literature includes numerous discussions about explosion behavior in interconnected vessels.
Experimental evidence has shown that explosions not only propagate, but become increasingly more damaging due to three phenomena [13]:
Flame Acceleration:
gas flow created by the primary explosion in a vessel will stretch the propagating flame into the pipes, increasing its surface area, its rate of combustion, and thus leading to higher flame speeds and pressure. Eventually, the initial deflagration (subsonic) can become a detonation (supersonic), resulting in much higher explosion pressures;
Flame Jet Ignition:
when the large and fast flame coming from the primary vessel will reach the secondary enclosure, it will ignite the remaining unburned material more violently and lead to higher explosion pressures and rates of pressure rise,
Pressure Piling:
gas expansion from the primary explosion will increase pressure into the pipes and the secondary enclosure (“pre-compression”) prior to the passage of the flame, leading to a more violent explosion than for ambient conditions.
Lunn et al. [14] carried out coal and toner dust explosions using a combination of contained vessels (2 m3, 4 m3, 20 m3), connected by a pipe up to 16 feet long. It was shown that an explosion in the primary vessel can result in explosion propagation and cause a much more violent explosion in the secondary vessel (both in terms of maximum rate of pressure rise and maximum explosion pressure, see Table 1). The same effect was shown, although to a lesser extent, for interconnected vented vessels by Holbrow et al. [9].
Primary Vessel (m3) |
Secondary Vessel (m3) |
Maximum Explosion Pressure (bar) |
2 |
20 |
8.4 |
20 |
20 |
11.4 |
2 |
2 |
14.7 |
20 |
4 |
19.7 |
Table 1. Summary of selected interconnected contained vessels explosion tests conducted by Health and Safety Laboratory (UK)
This aspect is also well illustrated by some of the worst dust explosions that ever occurred in grain elevators [15]:
1982 | Metz France
In 1982 (Metz, France), a dust explosion originated in a headhouse, spread throughout the upper gallery and spaces between silos (Figure 6)
Figure 6a. View of the Metz grain elevator before the explosion
Figure 6b. View of the Metz grain elevator after the explosion
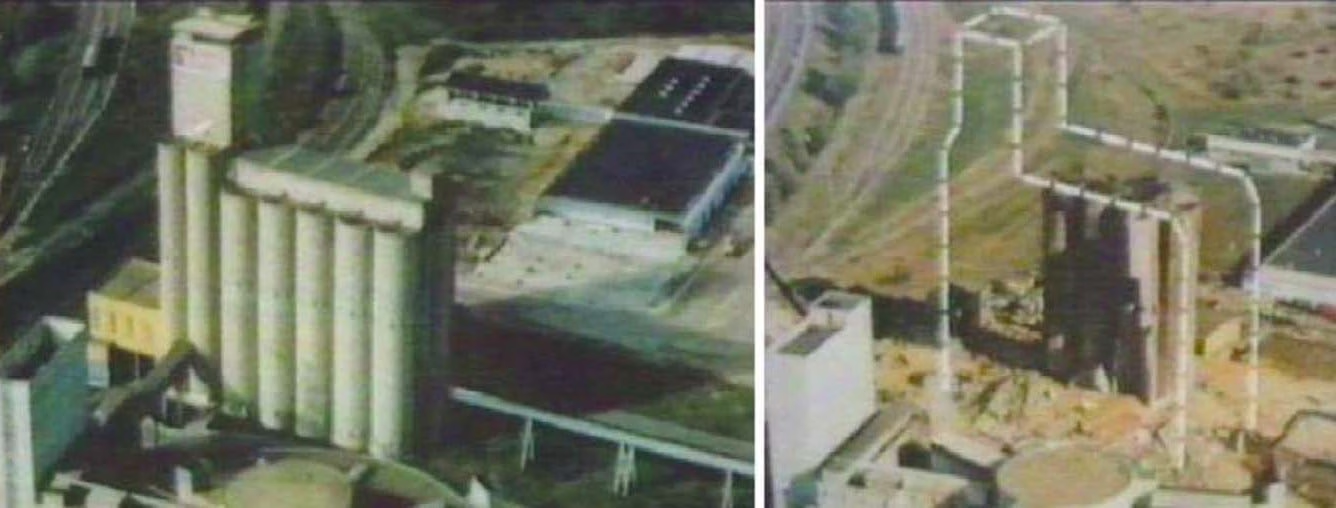
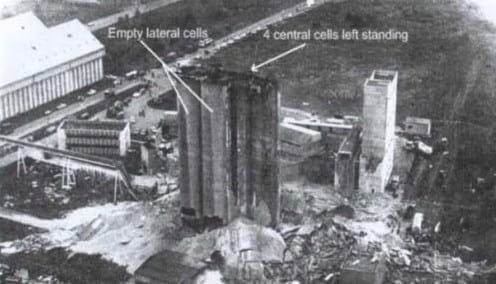
1997 | Blaye, France
In 1997 (Blaye, France), a dust explosion occurred in the northern headhouse before propagating into the upper gallery up to the southern end of the gallery (Figure 7)
Figure 7a. View of the damage caused by the Blaye explosion
Figure 7b. Schematic depiction of the supposed explosion at Blaye, France
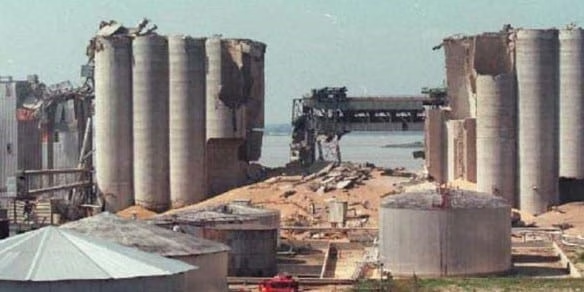
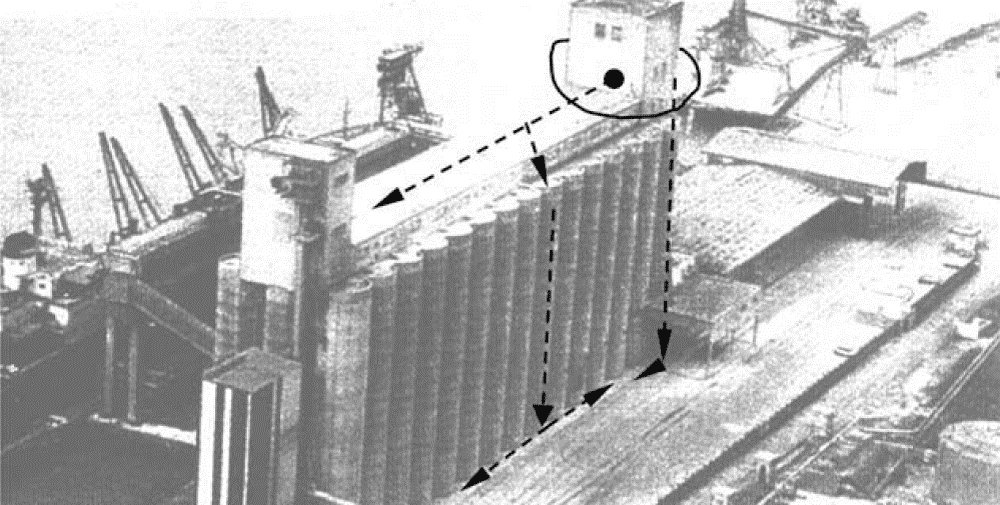
1998 | Wichita, Kansas, USA
In 1998 (Wichita, Kansas, USA), dust was ignited in the east tunnel of the south array of silos and propagated through the entire grain elevator (Figure 8)
Figure 8a. View of the DeBruce grain elevator after the explosion
Figure 8b. View of the DeBruce grain elevator after the explosion upper gallery
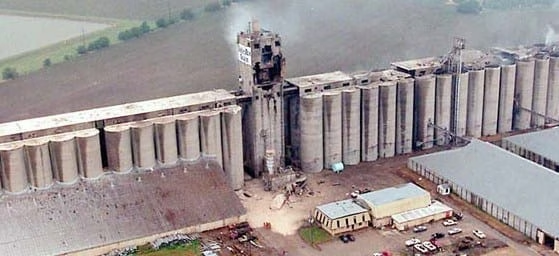
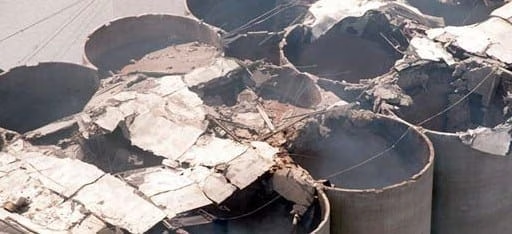
》 |
In all cases, a minor primary event was able to quickly develop into a major explosion involving an entire facility, leading to partial or entire collapse of reinforced concrete structures. |
myth
|
Small Diameter Pipes Do Not Support Dust Explosion Propagation |
fact |
Propagation is More Influenced by Pressure than by Pipe Diameter |
Small Pipes & Explosion Propagation
Dust explosion propagation in small pipes has always been a controversial topic. The primary argument being that flame propagation is challenged due to heat loss to the pipe walls.
In some of the experiments previously mentioned [11], Vogl used a 4 inch pipe and observed full propagation.
Van Wingerden et al. [8] observed different explosion propagation behaviors in 6 inch pipes, depending on the reactivity of the dust: while no propagation occurred with wheat dust (KST = 55 bar.m/s), maize starch (KST = 145 bar.m/s) was able to sustain full propagation in nearly all cases. They concluded that “the more reactive a dust is the higher the chance of flame propagation throughout the entire system is and the stronger the effect may be.” However, they also observed that “Dusts with similar KST values do not always show similar behavior when propagating through ducts”.
Andrews [16] conducted a comprehensive experimental program related to flame jet ignition of dust clouds. He investigated the effects of different parameters (primary vessel size and venting arrangement, dust, pipe diameter, pipe length, presence of obstacles) on the likelihood for a flame to propagate from a primary vessel and ignite a dust cloud in a secondary vessel. He alternatively used one 2 m3 vessel and one 20 m3 vessel connected by a 16 foot long pipe (6 inch, 10 inch, 20 inch diameter) to an 18.25 m3 vessel (flame jet ignition vessel).
While no flame transmission occurred using 6 inch pipe attached to the 2 m3 vessel, flame propagated in nearly all cases when using the 20 m3 vessel.
According to Fike Corporation’s research conducted with different fuels [5, 6], explosion propagation is more influenced by the reduced pressure and impulse developed in the primary vessel (whether generated by changes in KST, dust concentration or venting area), rather than by pipe diameter. Recent tests in Fike Corporation 5 m3 vessel-pipelines system using 6 inch pipes showed full propagation with and against process flow.
While conditions for dust explosions to propagate in relatively small diameter pipes are not yet fully established, their ability to propagate has been clearly demonstrated by several researchers.
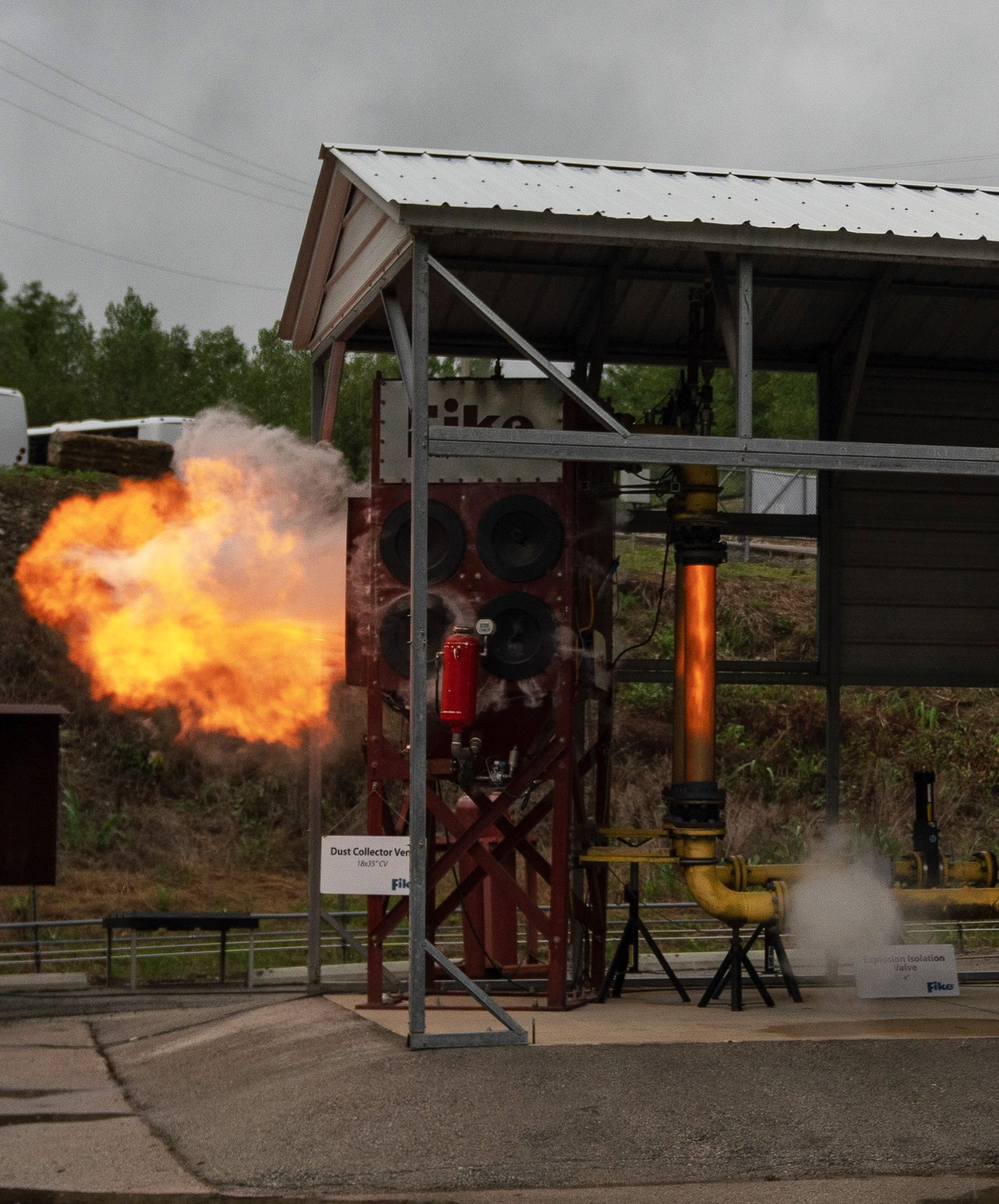
Conclusions
A review of past incidents and experiments reveals that dust explosion propagation in industrial-scale piping is a reality, and not a myth. History has shown that most devastating dust explosions occurred when the initial deflagration propagated and strengthened, causing widespread damage and numerous deaths.
While explosion protection techniques, such as venting and suppression, are generally applied to enclosures and equipment, the need for explosion isolation is underestimated because some myths about dust explosion propagation still remain. This article listed some of these myths and unraveled them.
Explosion propagation is affected by many parameters. Therefore, specific knowledge is of primary importance to determine the conditions under which explosion do or do not propagate.
Acknowledgments
The author acknowledges the invaluable efforts of Fike Corporation’s Explosion Protection team in conducting the flame propagation research. In addition, the author acknowledges the support of Fike Corporation for providing the resources and funding necessary to pursue this program and their permission to publish this work.